Researching the impact of hydrogen and ammonia on gas turbine component durability
The use of low-carbon fuels in power-generation gas turbines has attracted significant research and investment in recent years. There are several reasons hydrogen and ammonia have garnered so much interest as low-carbon fuels to blend with natural gas or eventually burn on their own to generate power.
A big driver, of course, is the potential to reduce power sector greenhouse gas emissions (GHG). For example, hydrogen produced using an electrolysis process powered by nuclear or renewable energy results in an ultra-low carbon fuel that can be burned in natural gas turbines, thereby dramatically lowering emissions. Additionally, as more intermittent renewable generation is added to the grid, increasing amounts of clean, firm, dispatchable electricity will be needed to maintain grid reliability when the sun isn’t shining and the wind isn’t blowing.
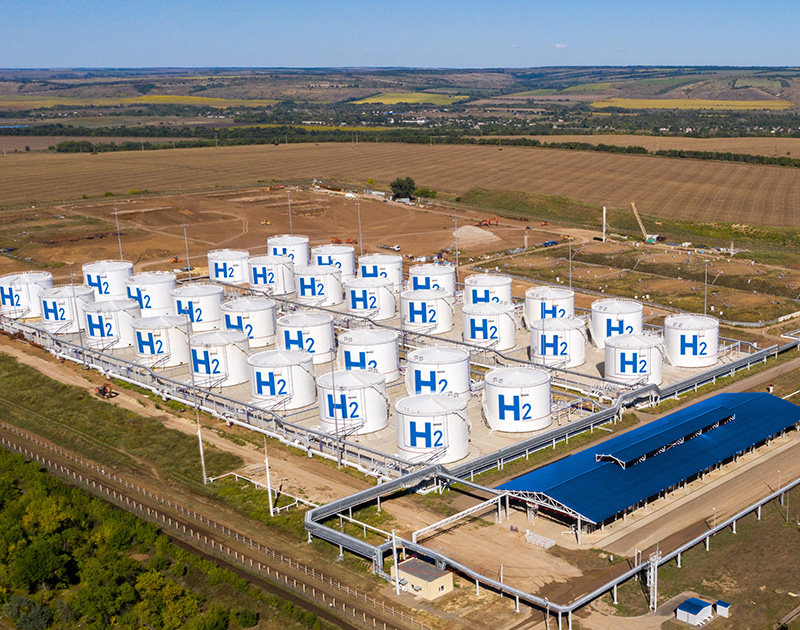
he U.S. Department of Energy (DOE) provided billions in funding to support the creation of a network of hydrogen hubs across the country to accelerate the production, storage, delivery, and end-use of hydrogen, including in power generation. Two California cities, Industry and Lancaster, announced the creation of the nation’s first public hydrogen utility in early 2025.
Initial Research Doesn’t Focus on Materials
Given its potential to drive decarbonization, numerous laboratory and field tests have been launched to probe the turbine performance and emissions reduction of hydrogen blended with natural gas. For example, EPRI’s Low-Carbon Resources Initiative (LCRI) partnered with utilities like Georgia Power, Constellation, and the New York Power Authority (NYPA) to evaluate co-firing gas turbines with fuels made up of 20 percent, 38 percent, and 44 percent hydrogen, respectively.
If low-carbon fuels are to be used more in gas turbines, however, a lot more needs to be understood about how they may potentially impact the components inside the turbine, especially in the so-called hot zone, where the combustion takes place. Because the components are exposed to such high temperatures, they tend to be the most expensive in the turbine. Hot-section components include the combustion liner, transition piece, and the vanes and buckets.
“As we think about hydrogen and other alternative fuels for low-carbon purposes, we mostly talk about burning it and whether the combustor can handle it and what the emissions are,” said Bobby Noble, an EPRI senior program manager whose work focuses on gas turbine research and development. “But there has been very little work on the implications for the rest of the hot section.”
An Important First Step to Understand Materials Impact
That is beginning to change. Last year, EPRI released “Preparing Gas Turbine Engine Materials for Low Carbon Fuels,” a review of research that had already been conducted and a suggested roadmap for future research to close knowledge gaps. Later this year, LCRI is also expected to publish the findings of tests of components with different coatings that have operated inside gas turbines for multiple years. The turbines used for the tests don’t burn hydrogen but instead have water injections for nitrous oxide (NOx) control that mimic fuel containing hydrogen.
The report released last year identified the elevated water content in the exhaust of gas turbines burning hydrogen or ammonia as a potential cause of accelerated material degradation. For example, when hydrogen burns, it reacts with oxygen, and water is the primary byproduct. Similarly, ammonia also produces water when it burns in a gas turbine. By contrast, when natural gas, which is primarily methane, burns in a gas turbine, it reacts with oxygen to produce both carbon dioxide and water. The water content present in the natural gas exhaust depends on the fuel’s composition and other factors.
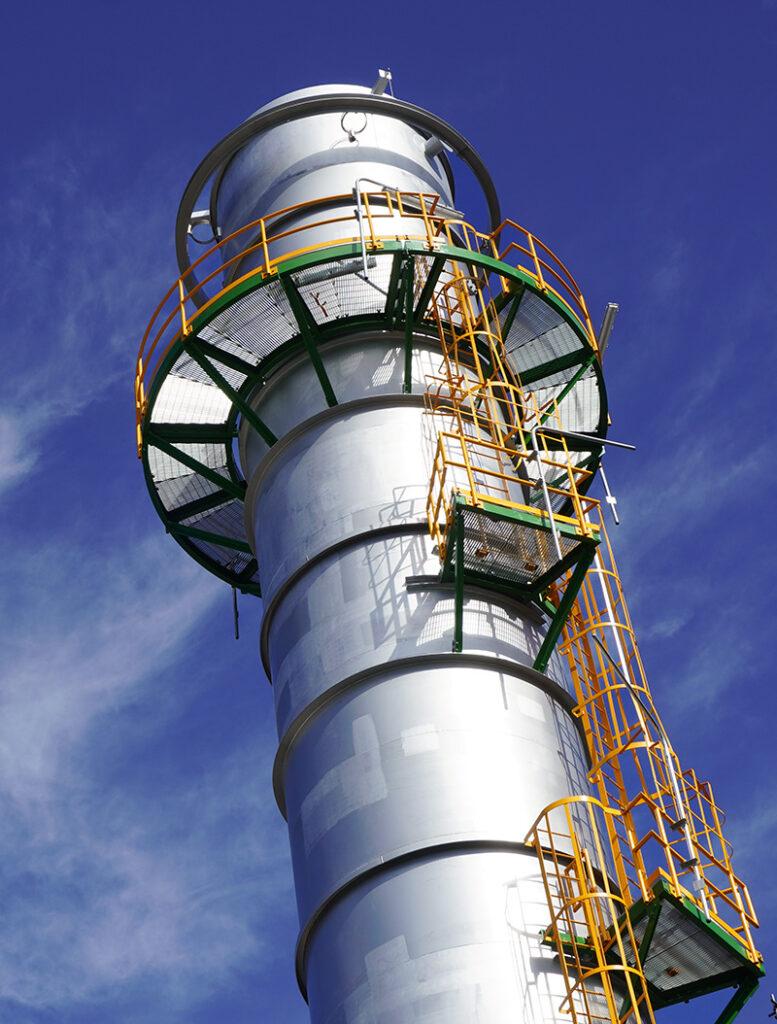
The Impact of Water Content
How does water content in a turbine’s combustion fuel exhaust impact the health and durability of components? A simple way to understand it is to imagine your own physical reaction to humidity, which measures the water content in the air. “70 degrees Fahrenheit with five percent humidity may be cold to some people. But 70 degrees Fahrenheit with 95 percent humidity is going to be miserable and sticky,” Noble said. “Similarly, having more water in the combustion exhaust in a turbine affects the heat transfer to the components. So even though the temperature of the fuel may be the same, the heat transfer can make the components hotter.”
The question is how much hotter components burning fuel mixtures with high water content can get and what extended exposure to elevated heat means for their expected useful lifetime. A greater understanding of the effectiveness of coatings and the turbine’s cooling system is also an important consideration when contemplating alternative fuels. “These components are designed to handle natural gas exhaust products,” Noble said. “The cooling schemes and everything else are highly tailored to keep the components to the right temperature under typical conditions for that fuel. Now that we are considering different fuels with higher water content, that design may or may not be sufficient.”
Other key findings of the report, which was spearheaded by Jacqueline O’Connor, professor of mechanical engineering at Penn State University, included:
- Past studies to understand the impact of higher water content exhaust on hot zone components were not done at relevant operating conditions and environmental conditions, making it difficult to estimate the impact of low-carbon fuels.
- Studies of material compatibility for high hydrogen fuels have mainly focused on fuel handling systems like piping and compression. While important, this is one piece of advancing long-duration operation of hydrogen in power generation gas turbines.
- Many additional research questions need to be investigated and answered.
The Road Ahead
There is a benchmark for how long components should last. There are always nuances in terms of which specific components and protective coatings are used and the type of turbine and fuel mix. However, in a general sense, components in the hot zone of a turbine running about 7,000 hours per year should last between four and six years.
Some refineries and chemical plants have gas turbines that have burned hydrogen for years. However, the components in the turbines are older technology, and there have not been any systematic studies comparing the impact of burning hydrogen and natural gas on their useful lifetimes. Modeling is currently the only way to gauge how new fuels may impact components.
That is now changing. Recently, EPRI and Penn State teamed up to begin laboratory testing of hot-zone components. The testing is being informed by input from LCRI and EPRI’s advanced gas turbine (Program 217) and materials program (Program 229). The testing will expose components to differing levels of heat and humidity for various periods of time. The components will also be subjected to destructive testing. “We want to build a materials database based on various scenarios,” Noble said.
In his discussions with utilities, Noble has found that there is a lot of interest in the test results. But it’s also clear that a lot more research will be necessary to fully understand how low-carbon fuels with high water content will affect the lifespan of hot-section components.
A wide range of potential research topics were laid out in the EPRI report released last year.
As the electric power industry considers greater use of low-carbon fuels, gas turbines must also evolve to handle the unique challenges of hydrogen, ammonia, and other alternatives. Continuing research into materials, coatings, and system performance is crucial to ensure turbines remain reliable as alternative fuels scale and become more viable options.
EPRI Technical Expert:
Bobby Noble
For more information, contact techexpert@eprijournal.com.