There is little debate about the urgent and growing need for large amounts of affordable energy storage. The many reasons energy storage is an essential pillar of a resilient, reliable, and decarbonized grid are well-known, particularly its role in supporting the installation of large amounts of intermittent renewable generation.
For example, the International Energy Agency (IEA) recently reported that global renewable electricity capacity additions surpassed 500 gigawatts in 2023 and would exceed 40 gigawatts in the U.S. alone in 2024. As renewable penetration increases, so does the need for storage. Indeed, the IEA projects that about 1,500 gigawatts of energy storage will need to be installed by 2030 under its net-zero emissions by 2050 scenario.
Though pumped hydro has long been the dominant energy storage technology, the need for low-cost, reliable, and flexible storage technologies has spawned significant investment and research and development (R&D). While much of the energy storage focus is on various battery technologies, it is increasingly clear that a diverse mix of short-and long-duration storage solutions will be needed to cost-effectively handle the growing number of storage use cases.
Growing Attention to Thermal Energy Storage
Over the past few years, thermal energy storage systems have attracted a lot of interest and been the focus of significant R&D. Earlier this year, the readers of MIT Technology Review chose thermal energy storage as one of the ten breakthrough technologies of 2024. That interest is expected to translate into meaningful deployments. The International Renewable Energy Agency (IRENA) forecast indicates that thermal energy storage technologies could triple in size by 2030, reaching 800 gigawatt-hours of installed capacity.
At the most basic level, thermal energy storage systems capture and store heat in materials like bricks, molten salt, and concrete for discharge later. An earlier EPRI Journal story detailed how concrete thermal energy storage technology works and its potential benefits, including providing a far cheaper and much longer-duration storage option than lithium-ion batteries. The story also chronicled laboratory research EPRI conducted with concrete thermal energy storage system developer Storworks Power that successfully validated the technology’s design and performance.
However, anybody involved with R&D knows that strong laboratory performance does not guarantee that a technology will work as expected in the real world. “There’s always a vision of what works from a modeling and lab point of view,” said Scott Hume, an EPRI technical executive whose research focuses on energy storage. “But ultimately, it’s only when you truly build and operate it that you start to see real challenges, or if the technology works as you hoped.”
The aim of a recently completed pilot project conducted by EPRI, Storworks, and Southern Company was to gain a realistic understanding of how concrete thermal energy storage functions in an operational utility setting. A 10-megawatt-hour concrete thermal energy storage system (CTES) was designed and constructed at Alabama Power’s Plant Gaston, a five-unit, 1880-megawatt natural gas and coal power plant in Wilsonville, Alabama. The CTES included 42 of Storworks’ concrete “Bolderbloc” units, each embedded with numerous stainless-steel tubes. The pilot project finished construction in the summer of 2023 and began commissioning and testing in the fall of the same year.
For Storworks Power, the project was an opportunity to test its CTES at a scale that is impossible to reach in the lab. “Storworks has done many lab-scale tests on different full-size CTES modules,” said Jennifer Tuey, the company’s director of R&D. “The Plant Gaston pilot project gave Storworks the opportunity to build a system more than 10 times larger and integrate with a live boiler at an operating facility.” Tuey says Storworks was eager to learn how its CTES operated as it was charged with superheated boiler steam and discharged to make superheat steam.
For Southern Company, one of the aims of the demonstration of the CTES at Plant Gaston’s Unit 5 was to understand better how this technology could support plant flexibility. “Our primary goals were to prepare for successful large-scale deployment via hands-on testing and evaluation, reduce cost and technology risk, and identify gaps and learn,” said Josh Barron, a senior research engineer at Southern Company, the parent company of Alabama Power. “The primary metrics we were interested in were roundtrip efficiency, thermal losses, and reliability.”
Enhancing Flexible Operations at Fossil Plants
In recent years, coal plants and other fossil fuel assets across the U.S. have had to operate in ways they weren’t initially designed for. As more wind and solar have been added to the grid, some coal plants have evolved from continuous baseload operation to more flexible load-following output. To operate with greater flexibility usually means a power plant must ramp up and down quickly to respond to changes in net demand.
EPRI has conducted extensive research about the potential strains that can result from flexibly operating a fossil fuel power plant originally designed for baseload generation. The CTES demonstration at Plant Gaston probed how thermal storage could help by accepting steam from the plant’s boiler when net demand was falling or quickly responding to increased net demand by generating steam. Discharging steam in response to increasing demand is especially helpful in regions with large amounts of solar, where total generation declines significantly as the sun sets. Among other performance metrics, the researchers at Southern Company monitored whether the temperature, flow rate, and duration of the CTES discharge could accurately mimic the typical supply to the turbine.
A Rigorous Testing Plan
The testing protocol for the CTES was designed to reflect myriad conditions. The CTES was charged at different rates to validate its capacity to store thermal energy and discharge it at differing rates. For example, while the need to respond to a setting sun and dropping solar generation by rapidly producing steam for the coal unit’s turbine is one important scenario, it’s not the only one. Also important is the ability to discharge steam to supply the turbine at a more modest pace but over a longer period.
The research and construction teams also had to navigate challenges well beyond the normal hurdles of a pilot project. Indeed, the project began during the COVID-19 pandemic, when access to the plant was limited to essential personnel. The project also faced supply chain constraints, resulting in cost increases due to the disruption caused by the pandemic. “The pandemic created significant challenges for the overall timeline of the project. The Southern Company and Alabama Power staff did an excellent job managing the construction team to get this project built,” Hume said. “Some of the special hardware, such as small valves needed for high pressure and temperature, arrived over a year after they were ordered. But Southern Company did a great job scheduling workers around when parts arrived.”
When construction of the CTES was finished, an eight-part testing plan began. The testing plan included:
- Baseline tests involved charging the CTES at full supply pressure for four to six hours and then discharging at the same nominal flow rate for four hours.
- Undercharge test in which the CTES was charged at the same rate as the baseline test but where the charging was stopped after two to three hours and then switched to discharge.
- Fast charge test involved delivering the maximum feed pressure at a higher than nominal flow rate to the CTES.
- Slow charge test charged the CTES at a deliberately slow flow rate.
- High power discharge test was designed to probe the system’s capacity to deliver the maximum quantity of steam. However, limitations with the condensate pump prevented the test from being completed.
- Slow discharge test evaluated the CTES’ ability to deliver steam over a prolonged period at partial load conditions.
- Variable-pressure discharge test investigated the possibility of changing the pressure of the steam released by the CTES to optimize the system’s capacity.
- High-pressure discharge test probed whether the CTES could produce higher-pressure steam that could be used for higher work potential.
Lessons Learned
The CTES at Plant Gaston completed 86 full charging and discharging cycles under a wide range of conditions. One important takeaway from the testing is that the CTES consistently and successfully demonstrated its ability to enhance the unit’s flexible operations without any adverse impacts on the plant and its components.
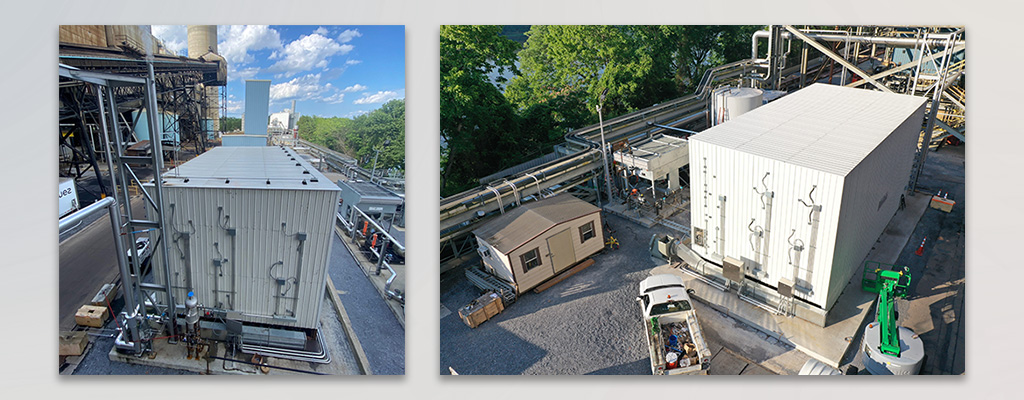
Enhanced flexibility was made possible by the reliable responsiveness of the CTES. “Storworks operated the CTES system 24/7 for three weeks, and the system responded immediately with changing pressures, temperatures, and flow rates at all states of charge and discharge,” Tuey said. “We were pleased that the system operated as expected and thermal performance matched our modeling. Another key takeaway is that at the end of a discharge, the discharge pressure can be lowered to access a ‘reserve’ of thermal energy that is available to extend the value of the system even if operating below rated conditions.”
Other specific lessons learned from the project include:
- The initial concrete drying and commissioning procedures were refined for future deployments to improve their efficiency.
- The flexibility of the constructed CTES exceeded expectations, and the CTES was able to respond consistently to changing conditions.
- Design improvements were identified to enhance system efficiency in future deployments. “For future iterations, more insulation is needed on the hot end of the blocks to reduce thermal losses,” Barron said. Barron also noted that consistent heat transfer between each row of blocks could be improved by installing control valves at each manifold.
- Full cycle thermal roundtrip efficiencies exceeded 88 percent, and Storworks believes efficiency can be significantly improved. “With improvements identified in the final project report, Storworks believes thermal roundtrip efficiencies will be more than 98 percent,” Tuey said.
- The experience of integrating CTES into an operating power plant provided Storworks with insights that can lower the costs of future projects. “Storworks has a better understanding of the processes for plant integration and costs of construction and operation of their system onsite,” Tuey said. “Storworks is very cost-conscious. Thus, we have refined these details and added reduction opportunities in our cost models.”
Looking Ahead
After any successful demonstration project, the question is always the same: What comes next? While there are no immediate plans to deploy CTES at scale in Southern Company’s system, Barron says there may be potential applications in the future.
“It will be an option to consider as renewable energy and the need for long-duration storage grow within our service territory,” Barron said. “One potential near-term application of CTES would be to deploy it at industrial customer sites to decarbonize high-temperature industrial heat. That was not an application we had initially considered when we started this demonstration project, but interest in this type of technology is growing among industrial customers.”
It’s not just utility industrial customers who are interested in CTES and other thermal storage technologies. One big reason industrial customers are exploring thermal storage is that they see it as a tool to help drive their decarbonization efforts. The size of the storage system needed at industrial sites also makes them more viable projects. “Industrial market deployments are smaller, so it’s easier to make a project happen because it’s a smaller use case,” Hume said. “A utility-scale storage system is on a gigawatt-hour scale whereas something on a petrochemical plant or a factory could be on the tens of megawatt-hour scale.”
Demonstration projects like the one at Plant Gaston are important for advancing technology and understanding gaps that need additional research and development. In fact, Southern Company’s Barron says more storage technology demonstrations are needed. “First-of-a-kind technology deployments are always difficult, but many more projects like this are needed to meet clean energy goals,” he said.
But it’s also important to remember that no single technology will be enough to meet the large and growing need for energy storage. And choosing the right storage technology will be determined in large part by its application, where it’s located, market conditions, and regulatory approval.
“Each technology has pros and cons in terms of performance and cost,” Hume said. “It’s relevant to your location, too. Are you in a location with a lot of nuclear power? Is it a highly renewable environment? If it’s a solar-dominated market, the storage durations and roundtrip efficiencies will be different than if it’s wind-dominated. CTES and thermal storage will be part of the answer. The industry, customers, and other stakeholders need to figure out when and where it should be deployed.”
EPRI Technical Expert:
Scott Hume